Cosmic Chemistry: The Mineral Miracle That Could Have Birthed Earth's First Life
Science
2025-04-12 22:45:03Content
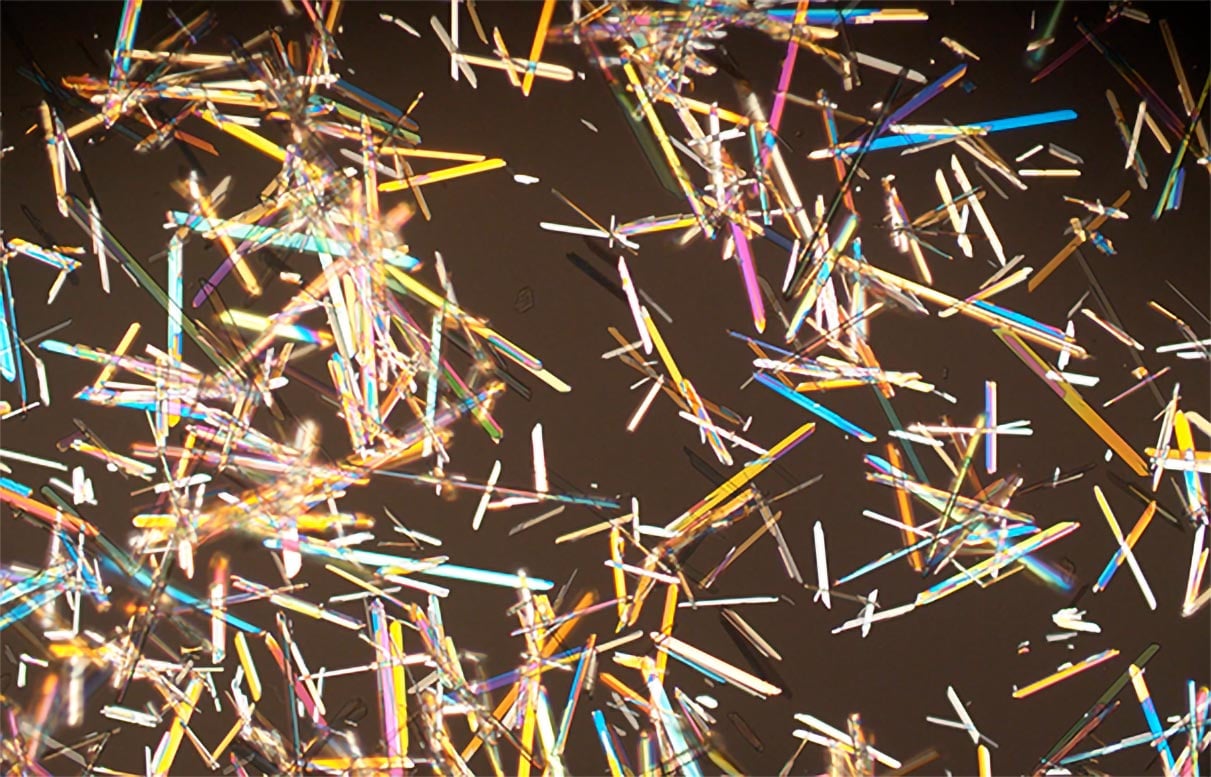
In a groundbreaking discovery, scientists have uncovered a fascinating potential mechanism behind the origins of molecular asymmetry in early life forms. Researchers from the Earth-Life Science Institute (ELSI) at the Tokyo Institute of Science have revealed how calcium might have been a crucial architect in determining the molecular handedness of primitive polyesters and fundamental biomolecules.
The study sheds new light on one of the most intriguing mysteries in prebiotic chemistry: how certain molecules developed their distinctive left or right-handed structural configurations. Calcium, typically known for its role in biological processes like bone formation, appears to have played a surprising and pivotal role in guiding molecular chirality during the early stages of chemical evolution.
This unexpected finding opens up exciting new avenues for understanding how the complex molecular building blocks of life might have first emerged and organized themselves, potentially providing critical insights into the fundamental processes that preceded the development of the first living systems.
Unraveling the Molecular Mysteries: How Calcium Shaped Early Life's Chemical Architecture
In the intricate landscape of scientific discovery, researchers continue to probe the fundamental origins of life, seeking to understand the complex chemical interactions that transformed simple molecular structures into the building blocks of biological systems. Recent groundbreaking investigations have shed light on a fascinating phenomenon that challenges our previous understanding of molecular evolution.Decoding the Chemical Foundations of Primordial Life Forms
The Calcium Catalyst: Molecular Handedness and Primitive Chemistry
Scientists at the Earth-Life Science Institute have uncovered a remarkable insight into the potential role of calcium in guiding molecular development during the earliest stages of chemical complexity. The research reveals an extraordinary mechanism by which calcium ions might have influenced the spatial orientation of molecular structures, particularly in primitive polyester-like compounds. At the microscopic level, molecules can exist in mirror-image configurations, a property known as chirality. This phenomenon is crucial in biological systems, where specific molecular orientations determine critical functional characteristics. The study suggests that calcium played a pivotal role in selecting and stabilizing particular molecular configurations, potentially setting the stage for more complex biochemical interactions.Crystalline Clues: Microscopic Evidence of Molecular Transformation
Advanced imaging techniques, including polarization microscopy, have provided unprecedented visual insights into the intricate world of molecular crystallization. Researchers observed how calcium tartrate crystals formed unique structural patterns, offering a window into the potential mechanisms of molecular self-organization. The microscopic landscapes revealed through these techniques demonstrate the nuanced interactions between calcium ions and organic molecules. Each crystal formation represents a complex dance of chemical affinities, where molecular components align and interact with remarkable precision.Implications for Understanding Prebiotic Chemical Evolution
This groundbreaking research extends far beyond mere academic curiosity. By understanding how fundamental chemical elements like calcium might have guided molecular development, scientists can reconstruct potential pathways of early life formation. The findings challenge existing models of chemical evolution and suggest a more intricate narrative of how life's molecular foundations were established. The role of calcium emerges as more than a passive participant in chemical processes. Instead, it appears to be an active director, potentially influencing molecular configurations that would ultimately contribute to the emergence of more complex biological structures. This perspective opens new avenues for exploring the origins of life's molecular complexity.Technological and Scientific Frontiers
The implications of this research stretch across multiple scientific disciplines. Researchers in fields ranging from biochemistry to astrobiology can leverage these insights to develop more sophisticated models of molecular interactions. The understanding of how simple ions like calcium might guide molecular self-organization could revolutionize our approach to studying prebiotic chemical systems. Moreover, the methodological innovations demonstrated in this study—particularly the advanced microscopic imaging techniques—provide powerful tools for investigating molecular interactions at unprecedented levels of detail. These technological advancements represent significant progress in our ability to probe the microscopic world and unravel its complex mechanisms.Future Research Directions
While the current findings are groundbreaking, they also highlight the vast unknowns in our understanding of molecular evolution. Future research will likely focus on expanding these observations, investigating how similar mechanisms might have operated across different chemical environments and exploring the broader implications for understanding life's origins. Interdisciplinary collaboration will be crucial in advancing these investigations. By combining expertise from chemistry, biology, geology, and computational modeling, researchers can develop more comprehensive frameworks for understanding the intricate chemical processes that preceded biological life.RELATED NEWS
Science
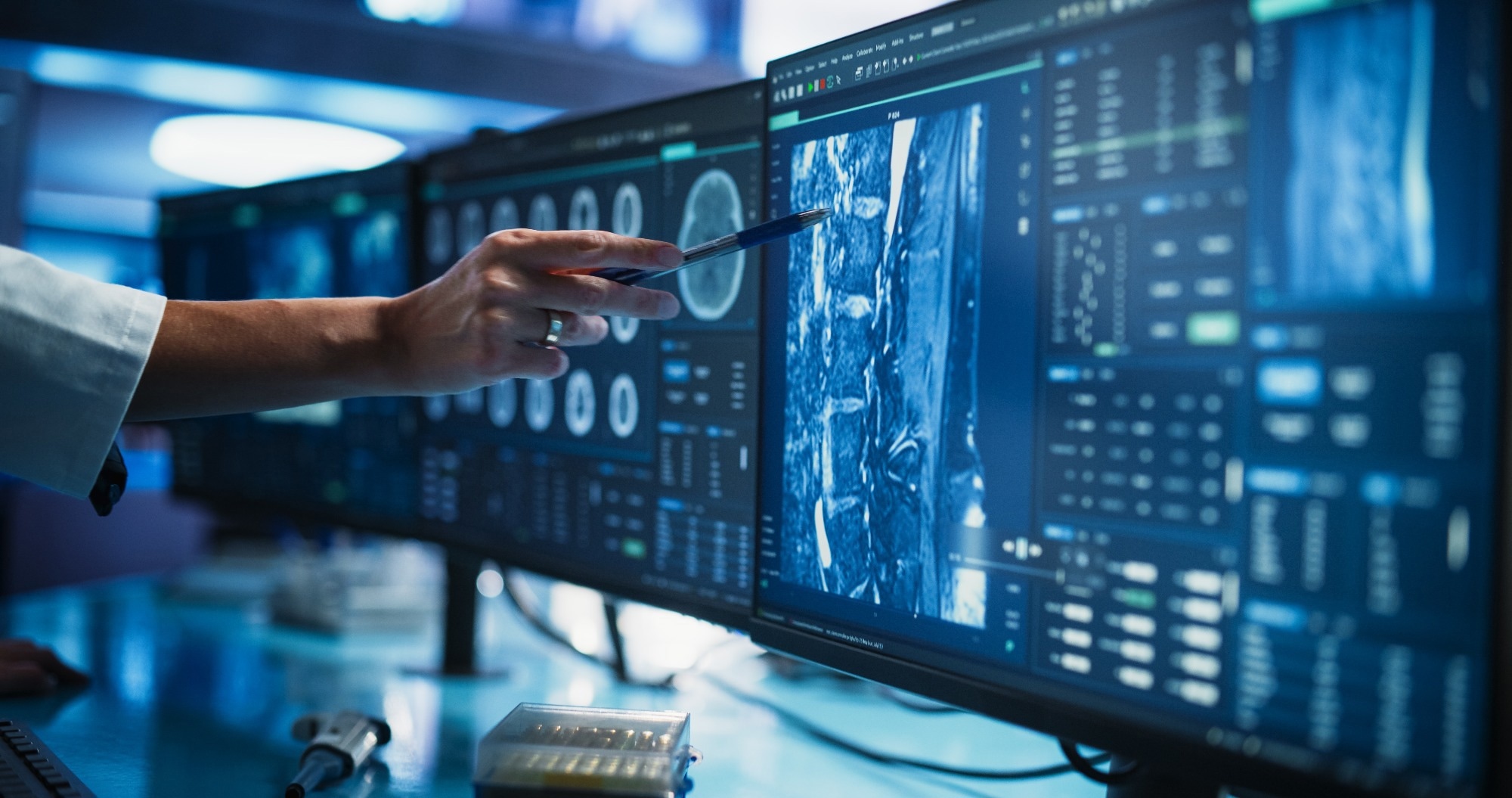
Breaking Barriers: How Bruker's Software Wizards Are Reshaping Scientific Innovation
2025-04-29 09:55:00
Science
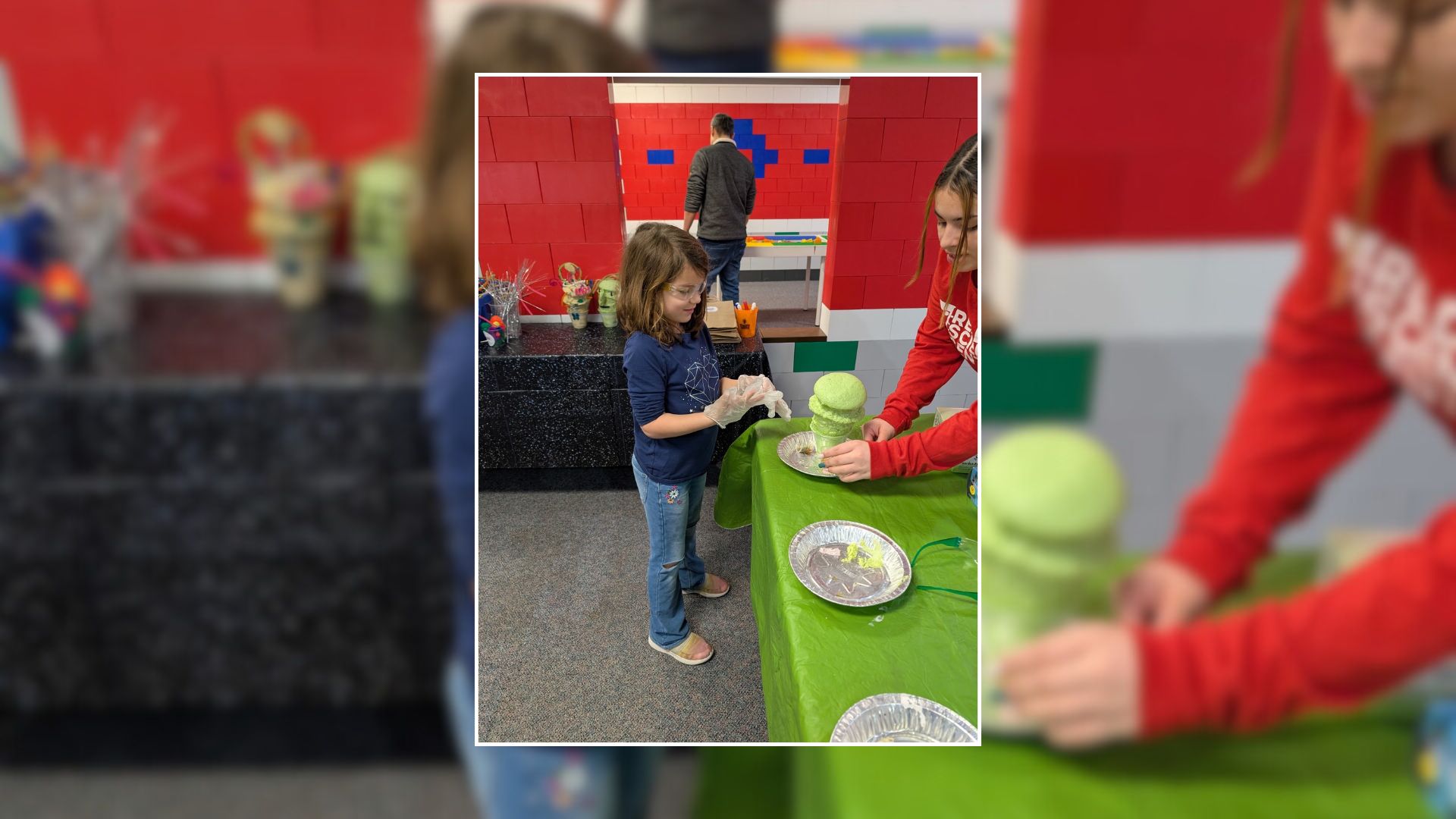
Community Builder: Local Realtor Fuels Science Center's Cutting-Edge Lab Transformation
2025-03-31 08:30:00
Science
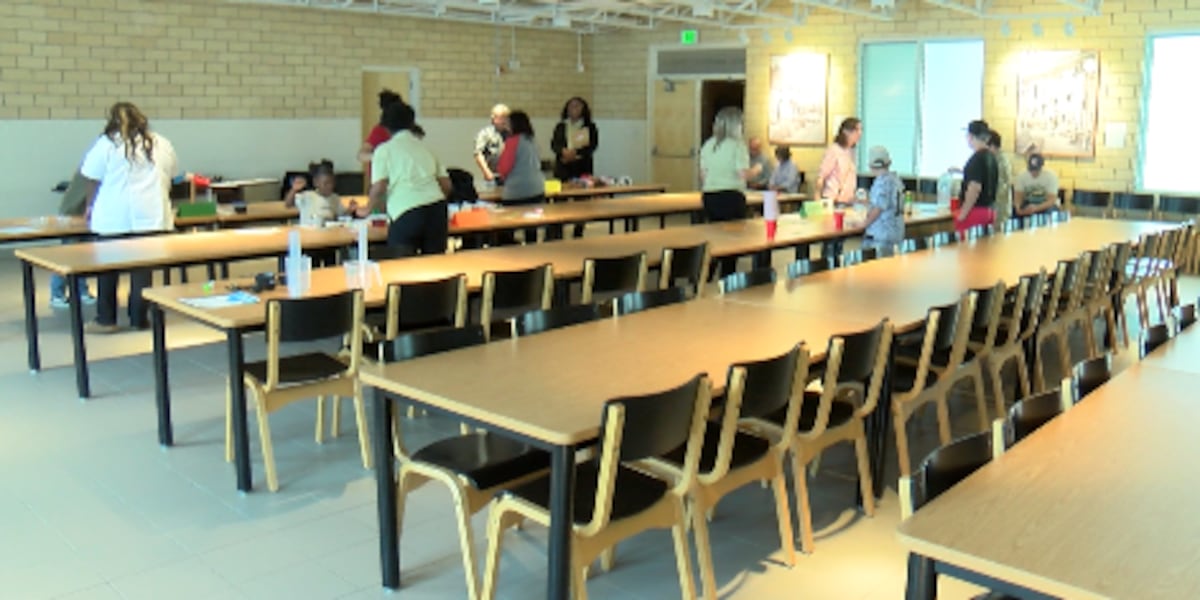
Unleashing Scientific Curiosity: Smith's Weekend Extravaganza Transforms 6th Street Museum District
2025-03-09 04:19:26